Esters – Functional Group Spotlight
Esters are such a ubiquitous and important functional group, and they’re often one of the first to be encountered in preparative chemistry labs, since they are so readily formed. Hot on the heels of his recent Acetals and Ketals Functional Group Spotlight, MedChemProf has returned to the world of carboxylic acid derivatives with this overview of Esters. Once again – be sure to try out the interactive 3D models! – Mark
The Ester functional group is a carboxylic acid derivative that is analogous to the Amides that were highlighted in Amide Functional Group Spotlight, but contain an Oxygen atom instead of a Nitrogen. While this may seem like a very small change, there are some important consequences for stability and reactivity which we will cover.
Ester Structure
Esters are ubiquitous in nature and are involved in many key metabolic processes, examples of which include the storage of fatty acids as triglycerides (fatty acid esters of the polyalcohol glycerol) or the transport of cholesterol as their lipophilic cholesterol esters. An example of a simple ester is exemplified in Figure 1 which shows Ethyl acetate (the ester that results from the combination of a molecule of Acetic acid and a molecule of Ethanol).
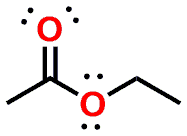
Figure 2 provides a look at Ethyl acetate as a 3D model instead of the flat 2D depiction in Figure 1.
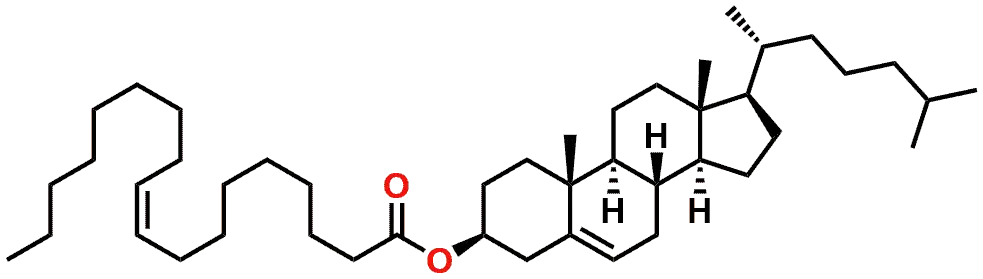
An example of a more complex ester is shown in Figure 3, which shows the Cholesterol ester resulting from the esterification of Cholesterol by the Fatty Acid Oleic acid.
Lactones are cyclic esters
As a side note, one piece of nomenclature that you may have also come across when learning about Esters is the term Lactone. A Lactone is simply an Ester that is part of a cyclic structure. A good example is the anti-cholesterol drug Simvastatin (aka Zocor). Shown in Figure 4, Simvastatin contains an ester (highlighted in the green box) as well as a 6-membered cyclic Ester (shown in the light blue circle). The cyclic Ester is typically referred to as a Lactone. The molecule can also be visualized in a 3D model in Figure 5.
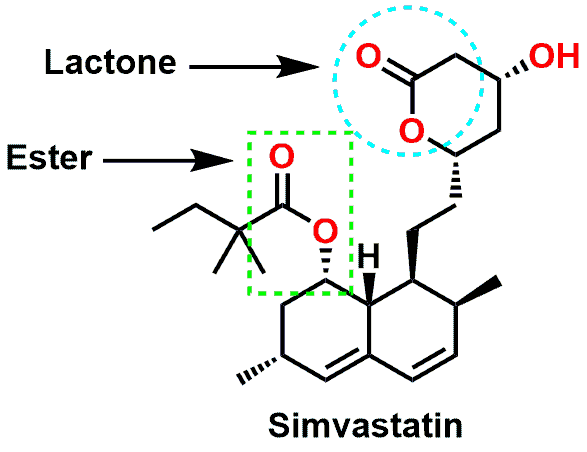
Ester Synthesis
Let’s continue our look at Esters by examining how they are formed and their general shape and bonding. Similar to Amides, Esters result from the overall dehydration of a Carboxylic Acid and an Alcohol (instead of an Amine) (see Figure 6). Please keep in mind that the reaction is reversible and that Esters are readily hydrolyzed under the right conditions to generate the corresponding carboxylic acid and Alcohol. This will become important later when we discuss the metabolism of drug molecules that contain esters.
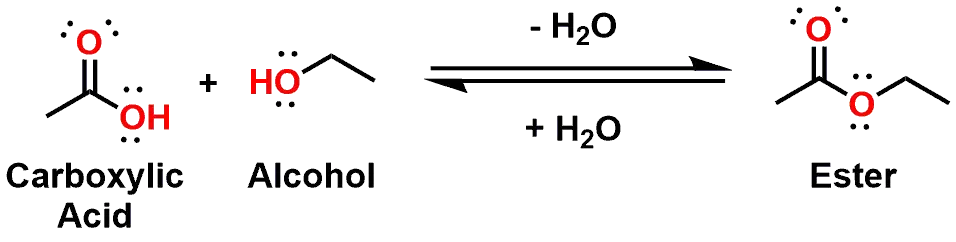
Ester Resonance Structures and Conformation
If we examine the shape and conformation of the Ester functional group, it will be noticed that one of the lone pair of the singly bonded Oxygen atom can be delocalized via resonance across itself as well as the Carbon and Oxygen atoms of the carbonyl group (C=O) (Figure 7).
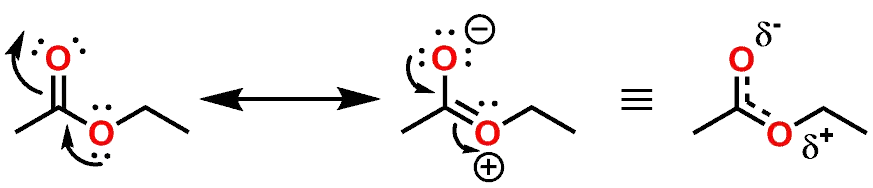
Due to the delocalization of the lone pair, the Oxygen (as well as the Carbon and Oxygen of the carbonyl) all must adopt an sp2 hybridization state. The lone pair delocalization and sp2 hybridization also imbues the atoms with a trigonal planar geometry. If the lone pair of the Oxygen atom was delocalized to the same extent as the lone pair of an Amide Nitrogen atom, you would assume that the Ester would be primarily locked into what is shown as the (E)- and (Z)-isomers (Figure 8).
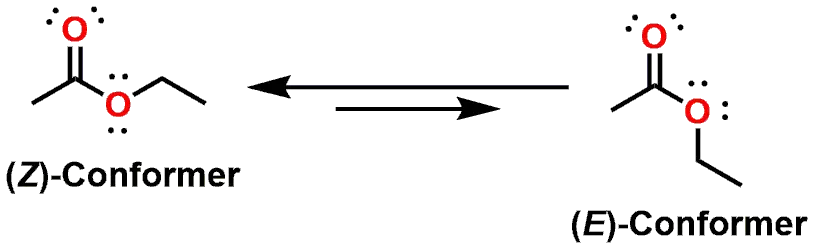
While these two forms are preferred energetically, it is not to the same extent as compared to that of an Amide. This means that the rotation around the Ester O-C=O bond is a lower energy process as compared to that of Amides. The greater flexibility and ease of rotation also makes Esters more volatile along with contributing to a lower melting points as compared to equivalent Amide structures. The additional flexibility also leads to greater water solubility as compared to Amides. Esters can act as Hydrogen Bond acceptors, but not as Hydrogen Bond donors.
Hydrolysis of Esters
Of primary concern in new Drug design is that Esters are more easily hydrolyzed than Amides. This is due to the increased electronegativity of O atom (versus the N of an amide) on the positive character of carbonyl carbon, thereby making it a better target for nucleophilic attack. Esters can be hydrolyzed under either basic conditions (such as by Hydroxide during saponification reactions), or under acidic conditions. Figure 9 shows the mechanism of the acid catalyzed hydrolysis of Ethyl acetate yielding an equivalent of Acetic acid and Ethanol as products.
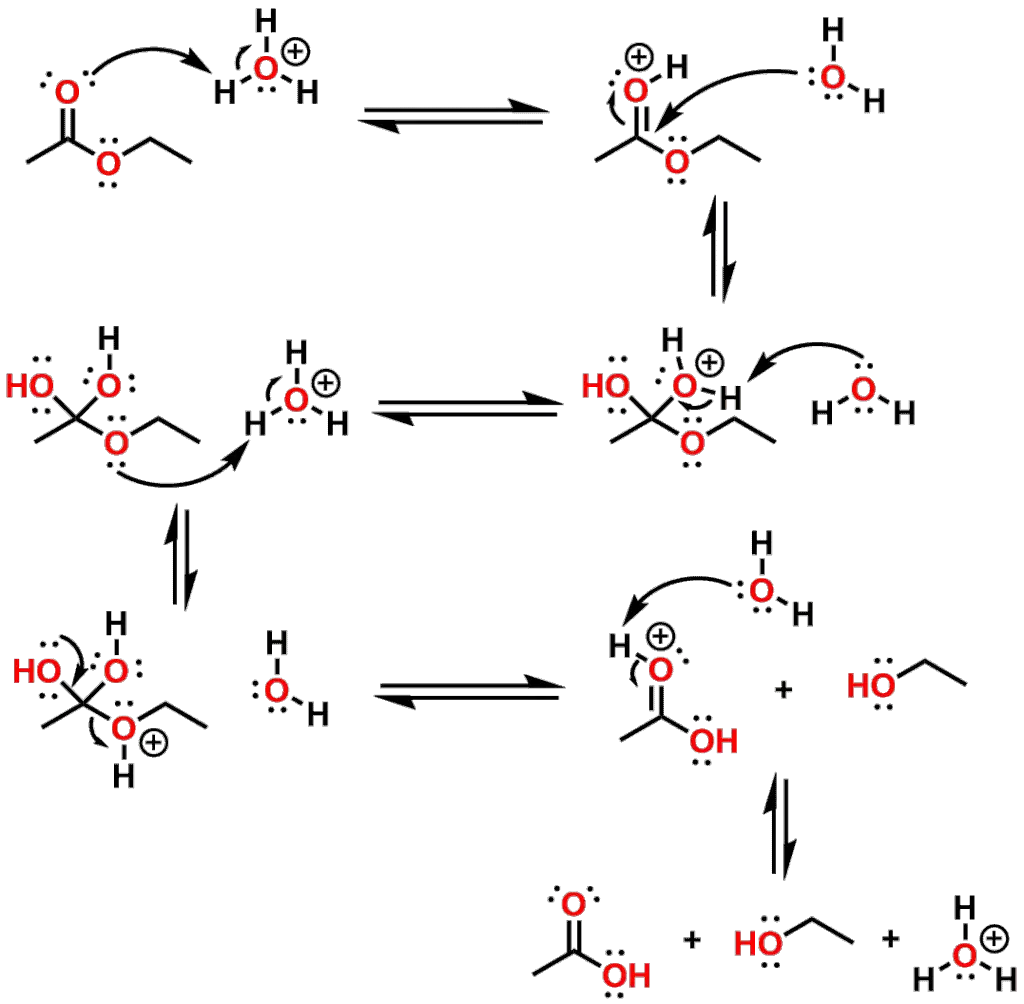
The Ester is first activated by protonation of the carbonyl oxygen of the Ester followed by nucleophilic attack by water. Subsequent to the attack by water, the central carbon of the Ester adopts sp3 hybridization and due to the tetrahedral shape it is known as the Tetrahedral Intermediate. While under acid catalyzed conditions, the Tetrahedral Intermediate is protonated, but under basic or neutral conditions, one of the Oxygen atoms would carry a negative charge. We will revisit this difference when we discuss the enzymatic hydrolysis of esters. In the below mechanism however, the Tetrahedral Intermediate then collapses to restore the carboxyl group as a Carboxylic acid with the corresponding Alcohol being expelled. All steps in the reaction mechanism are reversible and the relative concentration of the reactants will govern which direction the mechanism proceeds.
Enzymes that hydrolyze esters – Esterases
Esters are susceptible to hydrolysis from enzymes such as Esterases. These enzymes are typically equipped with an Active Site that contains three associated amino acid residues that facilitate the activation of the Ester, the nucleophilic attack of the ester, and proton shuttling to mediate the hydrolytic mechanism. Additionally, the Esterase enzymes also have amino acid residues situated properly to stabilize the negative charge of the Oxygen that is part of the Tetrahedral Intermediate. The location of the residues that stabilize and coordinate the oxygen of the anionic Tetrahedral Intermediate is known as the Anionic Hole.
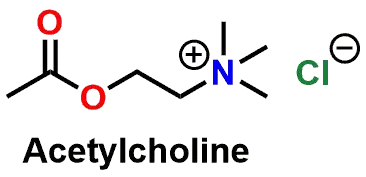
Hydrolysis of acetylcholine by acetylcholinesterase
The mechanism of the first part of the hydrolysis of the neurotransmitter Acetylcholine (Figure 10 and 3D Model in Figure 11) by the enzyme Acetylcholinesterase is shown in Figure 12. In the figure, it can be seen that the Serine hydroxyl group nucleophilically attacks the acetyl Ester of Acetylcholine to generate the Tetrahedral Intermediate.
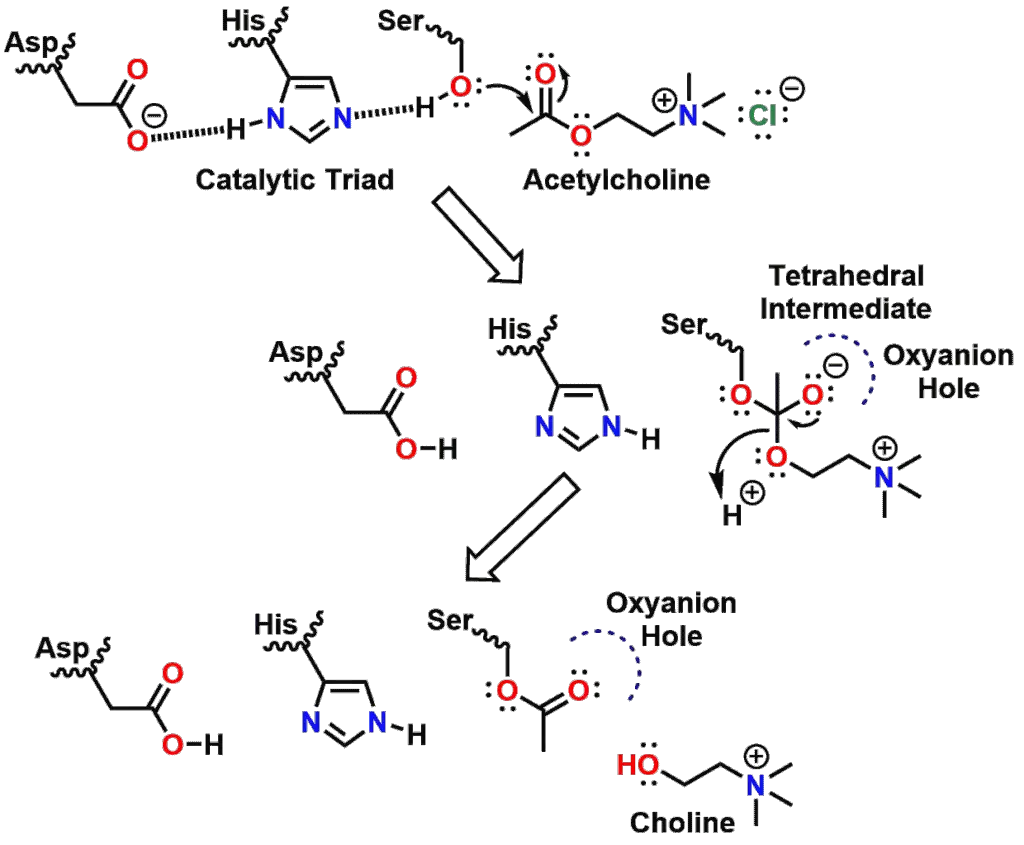
The 3D Model shown in Figure 13 highlights the Tetrahedral Intermediate of Acetylcholine hydrolysis, as well as the amino acid residues involved from Acetylcholinesterase. The Tetrahedral Intermediate then collapses to yield a molecule of Choline and the enzyme where the Serine residue has been acetylated. If Serine remained acetylated, then the enzyme would become inactive due to the covalent modification of one of the residues in the Catalytic Triad. A second step then occurs (not shown) whereby a molecule of water hydrolyzes the Serine ester under acid catalyzed conditions from the Aspartic acid. Once the Serine ester is hydrolyzed, the enzyme can then catalyze the hydrolysis of the ester of another molecule of Acetylcholine.
Esters in Drug Discovery
Since many Esters are rapidly targeted and hydrolyzed by esterase enzymes, their inclusion in new drug development candidates is often precluded due to concerns that they will be metabolized too quickly. While this scenario is not always true, the stability of any drug that contains an Ester needs to be evaluated carefully. The lability of the Ester functional group may also lead some to believe that they are not useful functional groups to include in drug molecules, but it turns out that the potential hydrolytic weakness can actually be exploited.
Ester pro-drugs
One example is with the drug Enalapril (see Figure 14) which is used to treat high blood pressure by inhibiting the enzyme Angiotensin-Converting Enzyme (ACE). It turns out that Enalapril cannot actually inhibit ACE, but must first undergo enzyme mediated Ester hydrolysis to produce the active metabolite known as Enalaprilat. The Carboxylic acid functional group is required to be present to be able to coordinate a Zn2+ ion in the active site of ACE. In this manner, Enalapril acts as what is known as a Prodrug, or a drug that is inactive until metabolized to produce an active molecule.
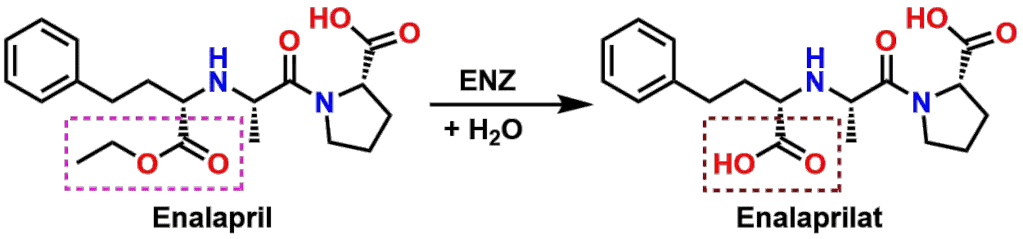
The reason that Enalaprilat cannot be used as a drug directly is that it is too poorly absorbed orally. The Ester form of the drug (Enalapril) however, is absorbed well orally, so it can be taken instead, followed by its enzymatic activation following absorption. As an interesting side note, the Lactone drug Simvastatin (shown earlier) is also a Prodrug. In order to be effective, the Lactone ring is required to be hydrolyzed to give the hydroxy-acid form of the molecule. Can you draw the hydrolysis product of the Lactone ring of Simvastatin?
Hey, it’s Mark here – I hope you enjoyed MedChemProfs introduction to Esters. If you liked this one, be sure to also check out his post on Amide Functional Groups.
Leave a comment below if you have any questions or to suggest the next topic for a Spotlight article.
Informative and comprehensive